The composites-intensive electric aircraft was purchased to meet the airline’s goal of flying a commercial demonstrator by 2026.
The $37 million contract will enable Piasecki to demonstrate its ARES tilt-duct VTOL aircraft and hydrogen fuel cell propulsion technologies. High Temperature Refractory
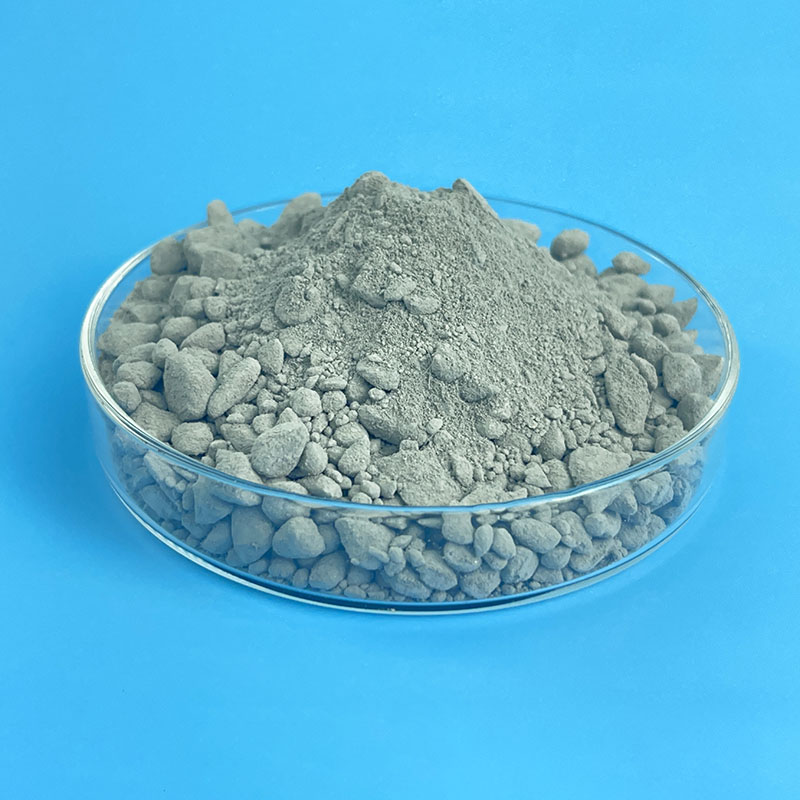
Design Organization Approval makes Lilium qualified to design and hold a type certificate for aircraft developed according to the EASA’s SC-VTOL safety objective rules.
The two-seat EL-2 Goldfinch is a blown-lift aircraft filling the gap for air travel routes between 50-500 miles. Certification and entry into service is targeted for 2028.
The Spanish electric mobility solutions developer is steadily growing its team and investments, emphasized by its rebranding from Umiles Next and the introduction of Integrity to customers.
$9.8 million will grow the eVTOL aircraft manufacturer’s footprint in Marina, California, support 690 new state jobs and accelerate early manufacturing to support initial commercial operations, targeted for 2025.
Evaluation of CFRTP m-pipe through Element’s U.K. facility aims to qualify the system for new operating environments.
Innovative prepreg tooling is highly drapable, capable of forming complex carbon fiber tooling shapes, in addition to reducing through thickness porosity and only requiring one debulk during layup.
Simutence and Engenuity demonstrate a virtual process chain enabling evaluation of process-induced fiber orientations for improved structural simulation and failure load prediction of a composite wing rib.
3D imaging and analysis capability illustrates detailed, quality characterization and performance simulation of composites and other advanced materials that properly captures the as-manufactured component.
Latest version of comprehensive simulation software speeds up computations and introduces surrogate model functionality.
As part of its efforts to automate as much of its production process as it can, Lyons Industries acquired a Massivit 10000 additive manufacturing system to quickly produce high-performance molds and support fixtures.
Qarbon Aerospace will focus on the design, development and manufacture of a thermoplastic composite structure for defense aviation components requiring icing protection.
Fraunhofer IFAM researchers and partners combine biodegradable polymer polycaprolactone and bioactive glass to 3D print custom-fit structures for bone fracture sites.
The carbon fiber wheel manufacturer and supplier has been awarded a total of 18 vehicle programs over the last couple of years, and is now looking to build capacity.
Lehvoss’ carbon fiber-reinforced thermoplastic composite materials are being used in the production of an e-bike set that will taken an expedition across North Africa.
Through the use of EzCiclio low-dielectric epoxy hardener, PCB manufactures can make recyclable, reusable products for electronic applications.
Terrene 3.0 is a compression-dominant arch developed as a multidisciplinary research project composed of natural fiber-reinforced sand and other biomaterials that delivers eco-friendly, less energy-intensive building methods.
New developments regarding productivity, maintenance and ergonomics make this enhanced composite placement system well suited for the production of complex parts.
Data collected from one year of outdoor testing reasserts the 3D-printed, bio-based structure’s viability to address housing challenges, sets the stage for future development.
Using Mechnano’s D’Func process, the new masterbatch of ogolimers enables 3D printing resin development with improved mechanical and nano-uniform electrical performance.
Collins Aerospace draws on global team, decades of experience to demonstrate large, curved AFP and welded structures for the next generation of aircraft.
Discussion of the issues in our understanding of thermoplastic composite welded structures and certification of the latest materials and welding technologies for future airframes.
The open AM innovation program welcomes all interested parties who would like to make use of the potential of 3D printing FGF or combine it with materials like carbon fiber, UD tape and other hybrid technologies.
CW explores key composite developments that have shaped how we see and think about the industry today.
Knowing the fundamentals for reading drawings — including master ply tables, ply definition diagrams and more — lays a foundation for proper composite design evaluation.
As battery electric and fuel cell electric vehicles continue to supplant internal combustion engine vehicles, composite materials are quickly finding adoption to offset a variety of challenges, particularly for battery enclosure and fuel cell development.
Performing regular maintenance of the layup tool for successful sealing and release is required to reduce the risk of part adherence.
Increasingly, prototype and production-ready smart devices featuring thermoplastic composite cases and other components provide lightweight, optimized sustainable alternatives to metal.
Interest in higher performance and more sustainability drive new composite materials innovations in sporting goods and other consumer products.
Manufacturers often struggle with production anomalies that can be traced back to material deviations. These can cause fluctuations in material flow, cooling, and cure according to environmental influences and/or batch-to-batch variations. Today’s competitive environment demands cost-efficient, error-free production using automated production and stable processes. As industries advance new bio-based, faster reacting and increased recycled content materials and faster processes, how can manufacturers quickly establish and maintain quality control? In-mold dielectric sensors paired with data analytics technology enable manufacturers to: Determine glass transition temperature in real time Monitor material deviations such as resin mix ratio, aging, and batch-to-batch variations throughout the process Predict the influence of deviations or material defects during the process See the progression of curing and demold the part when the desired degree of cure, Tg or crystallinity is achieved Document resin mix ratios using snap-cure resins for qualification and certification of RTM parts Successful case histories with real parts illustrate how sensXPERT sensors, machine learning, and material models monitor, predict, and optimize production to compensate for deviations. This Digital Mold technology has enabled manufacturers to reduce scrap by up to 50% and generated energy savings of up to 23%. Agenda: Dealing with the challenge of material deviations and production anomalies How dielectric sensors work with different composite resins, fibers and processes What is required for installation Case histories of in-mold dielectric sensors and data analytics used to monitor resin mixing ratios and predict potential material deviations How this Digital Mold technology has enabled manufacturers to optimize production, and improve quality and reliability
SolvaLite is a family of new fast cure epoxy systems that — combined with Solvay's proprietary Double Diaphragm Forming technology — allows short cycle times and reproducibility. Agenda: Application Development Center and capabilities Solutions for high-rate manufacturing for automotive Application examples: battery enclosures and body panels
OEMs around the world are looking for smarter materials to forward-think their products by combining high mechanical performance with lightweight design and long-lasting durability. In this webinar, composite experts from Exel Composites explain the benefits of a unique continuous manufacturing process for composites profiles and tubes called pull-winding. Pull-winding makes it possible to manufacture strong, lightweight and extremely thin-walled composite tubes and profiles that meet both demanding mechanical specifications and aesthetic needs. The possibilities for customizing the profile’s features are almost limitless — and because pull-winding is a continuous process, it is well suited for high volume production with consistent quality. Join the webinar to learn why you should consider pull-wound composites for your product. Agenda: Introducing pull-winding, and how it compares to other composite manufacturing technologies like filament winding or pultrusion What are the benefits of pull-winding and how can it achieve thin-walled profiles? Practical examples of product challenges solved by pull-winding
Composite systems consist of two sub-constituents: woven fibers as the reinforcement element and resin as the matrix. The most commonly used fibers are glass and carbon, which can be processed in plane or satin structures to form woven fabrics. Carbon fibers, in particular, are known for their high strength/weight properties. Thermoset resins, such as epoxies and polyurethanes, are used in more demanding applications due to their high physical-mechanical properties. However, composites manufacturers still face the challenge of designing the right cure cycles and repairing out-of-shelf-life parts. To address these issues, Alpha Technologies proposes using the encapsulated sample rheometer (premier ESR) to determine the viscoelastic properties of thermosets. Premier ESR generates repeatable and reproducible analytical data and can measure a broad range of viscosity values, making it ideal for resins such as low viscous uncured prepreg or neat resins as well as highly viscous cured prepregs. During testing, before cure, cure and after cure properties can be detected without removing the material from the test chamber. Moreover, ESR can run a broad range of tests, from isothermal and non-isothermal cures to advanced techniques such as large amplitude oscillatory shear tests. During this webinar, Alpha Technologies will be presenting some of the selected studies that were completed on epoxy prepreg systems utilizing ESR and how it solves many issues in a fast and effective way. It will highlight the advantages of this technique that were proven with the work of several researchers. Moreover, Alpha Technologies will display part of these interesting findings using the correlations between the viscoelastic properties such as G’ and mechanical properties such as short beam shear strength (SBS).
Surface preparation is a critical step in composite structure bonding and plays a major role in determining the final bonding performance. Solvay has developed FusePly, a breakthrough technology that offers the potential to build reliable and robust bonded composite parts through the creation of covalently-bonded structures at bondline interface. FusePly technology meets the manufacturing challenges faced by aircraft builders and industrial bonding users looking for improved performance, buildrates and lightweighting. In this webinar, you will discover FusePly's key benefits as well as processing and data. Agenda: Surface preparation challenges for composite bonding FusePly technology overview Properties and performance data
The incorporation of EMI shielding into composites is necessary in a wide range of applications — such as electronics and battery enclosures for AAM, automotive and aerospace — where EMI could interfere with the operation of the device, vehicle or aircraft, ultimately compromising security and control. TFP’s conductive nonwoven materials provide a solution, possessing a combination of properties that make them highly infusible, flexible, lightweight and an effective EMI shield. This combination allows them to overcome challenges in both application and process that more traditional substrates such as films, foils and paints struggle to achieve. In this webinar, Dr. Mark James will introduce TFP’s conductive nonwovens, their lightweight structure and EMI shielding capability. He will discuss how they are easily incorporated into composites to impart this functionality to the surface of a part, with some typical examples. Mike Campbell and Adam Halsband will then provide a case study on a new development for TFP materials as an EMI enhanced SMC compound. This compound is designed as a scalable, cost-effective solution for high throughput BEV applications, such as battery enclosures. Agenda: An introduction to TFP’s conductive nonwovens, their structure and manufacture The key physical properties and how they are tailored to suit end-use requirements How conductive nonwovens can be used effectively in a variety of applications A case study on the development and use of TFP’s veils in an EMI enhanced SMC compound for BEV applications
ICERP India is an important event of the Indian composites industry organized by FRP Institute once in every two years and ICERP event is the biggest event on Composites in India and second biggest event in Asia.
The annual Conference on Composites, Materials, and Structures (also known as the Cocoa Beach Conference) is the preeminent export controlled and ITAR restricted forum in the United States to review and discuss advances in materials for extreme environments. The Conference started in the 1970s as a small informal gathering for government and industry to share information on programs and state-of-the-art technology. Attendance has grown to nearly 500 people while preserving this same objective to share needs and trends in high-temperature and extreme environment materials, and the latest information on advanced materials and manufacturing processes. The five-day conference program includes two to three parallel sessions per day on topics including thermal protection materials, ceramic matrix composites, carbon-carbon materials, ballistic technologies, hypersonics, and gas turbine engines. Attendees are engineers, scientists, managers, and operational personnel from the turbine engine, aviation, missiles and space, and protective equipment communities. These communities include the Navy, Air Force, Army, MDA, NASA, DARPA, FAA, DOE, engine manufacturers, missile and aircraft manufacturers, commercial space companies, and material and component suppliers. The Conference will be held in St. Augustine again for 2024! Participation is limited to U.S. Citizens and U.S. Permanent Residents only with an active DD2345 certification.
The 48th International Conference & Exposition on Advanced Ceramics & Composites (ICACC 2024) will be held from Jan. 28–Feb. 2, 2024, in Daytona Beach, Fla. It is a great honor to chair this conference, which has a strong history of being one of the best international meetings on advanced structural and functional ceramics, composites, and other emerging ceramic materials and technologies.
The Transformative Vertical Flight (TVF) 2024 meeting will take place Feb. 6–8, 2024 in Santa Clara, California, in the heart of Silicon Valley and will feature more than 100 speakers on important progress on vertical takeoff and landing (VTOL) aircraft and technology.
The Program of this Summit consists of a range of 12 high-level lectures by 14 invited speakers only. Topics are composite related innovations in Automotive & Transport, Space & Aerospace, Advanced Materials, and Process Engineering, as well as Challenging Applications in other markets like Architecture, Construction, Sports, Energy, Marine & more.
JEC World in Paris is the only trade show that unites the global composite industry: an indication of the industry’s commitment to an international platform where users can find a full spectrum of processes, new materials, and composite solutions.
Thousands of people visit our Supplier Guide every day to source equipment and materials. Get in front of them with a free company profile.
Initial demonstration in furniture shows properties two to nine times higher than plywood, OOA molding for uniquely shaped components.
The composite tubes white paper explores some of the considerations for specifying composite tubes, such as mechanical properties, maintenance requirements and more.
Foundational research discusses the current carbon fiber recycling landscape in Utah, and evaluates potential strategies and policies that could enhance this sustainable practice in the region.
In its latest white paper, Exel navigates the fire, smoke and toxicity (FST) considerations and complexities that can influence composites design.
New white paper authored by Eike Langkabel, Sebastian de Nardo, and Jens Bockhoff, examines the best resin formulations for composites used in automotive part production, both structural parts and body panels.
Tension control plays a vital role in composites manufacturing in order to achieve automated processing, continuous processing, reduced scrap, increased product quality, and more, says a new white paper released by The Montalvo Corp.
Austrian research institute Wood K plus makes 95% silicon carbide ceramics more sustainable (>85% bio/recycled content), enables 3D shapes via extrusion, injection molding and 3D printing.
Thermoplastic polymer resin was designed to tackle distinctive industry challenges of large-scale 3D printing while also assisting with sustainability initiatives.
The MB9, representing a combination of high performance and eco-conscious materials use, will be commercially available in time for the 2024 sailing season.
For 42 months, the Aitiip Technology Center will coordinate the EU-funded project to design a new range of intermediate materials, such as pellets or resin-impregnated carbon fibers, which will be used to manufacture more sustainable final products.
Co-located R&D and production advance OOA thermosets, thermoplastics, welding, recycling and digital technologies for faster processing and certification of lighter, more sustainable composites.
The German Institutes of Textile and Fiber Research are targeting more sustainable carbon fiber via low-pressure stabilization and bio-based precursors, and working with Saint-Gobain to commercialize oxide ceramic fibers for CMC.
The composites industry is increasingly recognizing the imperative of sustainability in its operations. As demand for lightweight and durable materials rises across various sectors, such as automotive, aerospace, and construction, there is a growing awareness of the environmental impact associated with traditional composite manufacturing processes.
Closed mold processes offer many advantages over open molding. This knowledge center details the basics of closed mold methods and the products and tools essential to producing a part correctly.
During CW Tech Days: Thermoplastics for Large Structures, experts explored the materials and processing technologies that are enabling the transition to large-part manufacturing.
CW’s editors are tracking the latest trends and developments in tooling, from the basics to new developments. This collection, presented by Composites One, features four recent CW stories that detail a range of tooling technologies, processes and materials.
In the Automated Composites Knowledge Center, CGTech brings you vital information about all things automated composites.
CompositesWorld’s CW Tech Days: Infrastructure event offers a series of expert presentations on composite materials, processes and applications that should and will be considered for use in the infrastructure and construction markets.
Explore the cutting-edge composites industry, as experts delve into the materials, tooling, and manufacturing hurdles of meeting the demands of the promising advanced air mobility (AAM) market. Join us at CW Tech Days to unlock the future of efficient composites fabrication operations.
CompositesWorld’s CW Tech Days: Infrastructure event offers a series of expert presentations on composite materials, processes and applications that should and will be considered for use in the infrastructure and construction markets.
Explore the cutting-edge composites industry, as experts delve into the materials, tooling, and manufacturing hurdles of meeting the demands of the promising advanced air mobility (AAM) market. Join us at CW Tech Days to unlock the future of efficient composites fabrication operations.
Thermoplastics for Large Structures, experts explored the materials and processing technologies that are enabling the transition to large-part manufacturing.
MVP's Automated Equipment: Revolutionizing Composites Part Production Through Filament Winding within CompositesWorld's CompositesWorld Collections Knowledge Center
Composites One Offers Manufacturing Efficiencies with Aerovac Kitting Solutions within CompositesWorld's CompositesWorld Collections Knowledge Center
A report on the demand for hydrogen as an energy source and the role composites might play in the transport and storage of hydrogen.
This collection features detail the current state of the industry and recent success stories across aerospace, automotive and rail applications.
This collection details the basics, challenges, and future of thermoplastic composites technology, with particular emphasis on their use for commercial aerospace primary structures.
This collection features recent CW stories that detail a range of tooling technologies, processes and materials.
CMC is expanding, with new fiber production in Europe, faster processes and higher temperature materials enabling applications for industry, hypersonics and New Space.
CMC scales for growing, faster, hotter applications. GE Aerospace has pushed CMC production to new levels (top left) to meet aviation’s need for faster, more efficient engines. Other growing markets include space, for parts like rocket nozzles (lower left), and hypersonic vehicles, like those being commercialized by Hypersonix (lower right). To meet this demand, faster processing is maturing, such as MATECH’s FAST sintering (top center) used to densify C/SiC and SiC/SiC CMC in <10 minutes. CMC fiber is also being produced in Europe, such as DITF’s OxCeFi fibers (top right), successfully braided and tested in OCMC parts and being commercialized to industrial scale by Saint-Gobain. Photo Credit (top left, clockwise): GE Aerospace, MATECH, DITF, Hypersonix and ©DLR-WF via the Ceramic Network Landscape photo, top: Filament wound CMC parts produced by Schunk Kohlenstofftechnik (Heuchelheim, Germany). Photo Credit: Schunk, Ceramic Composites network/Composites United
- Industrial production in Germany - Growing use in aeroengines - Hypersonix - FAST: 99% dense CMC in <10 minutes - Laser CVD, short SiC fibers - U.K.’s “Ceramic Valley” and NCC - New CMC developments - Continuous fiber coating - Needs for expanded CMC future
Ceramic matrix composites (CMC) use ceramic fibers in a ceramic matrix to enable high-performance structures at high temperatures. For example, the silicon carbide (SiC) fiber-reinforced SiC matrix (SiC/SiC) CMC that GE Aerospace (previously GE Aviation, Evendale, Ohio, U.S.) produces for LEAP engine turbine shrouds can withstand 1,300°C, providing much higher resistance than metal superalloys like Inconel, but at one-third the density. This unique combination of properties has helped the LEAP engine run hotter with less cooling, improving efficiency to burn 15-20% less fuel, with lower emissions and maintenance. The GE9X engine, with five CMC parts, will reportedly be the most fuel-efficient engine ever built for a commercial aircraft when the Boeing 777X enters service in 2025.
Meanwhile, supersonic (Mach 1-5), hypersonic (Mach 5-10) and high-hypersonic (Mach 10-25) vehicles are in development that may need CMC not just in the engines but also in the airframes. For example, due to air friction from traveling at Mach 5, the nose cone and leading edges of such vehicles can see temperatures up to 1,600-2,800°C. R&D into ultra-high temperature CMC (UHTCMC) is aiming for service temperatures as high as 3,500°C.
CMC is also increasingly being sought for use in nuclear energy and other power generation plants (e.g., gas turbine, solar, hydrogen) as well as for thermal processes in industry. Again, higher temperatures increase efficiency. For example, prototype CMC reaction vessels for steam cracking hydrocarbons have shown as much as 50-60% improvement. However, there has been a limited supply of aluminum oxide (Al2O3) fibers used to reinforce the oxide matrix in such OCMC applications, says Denny Schüppel, managing director for the Ceramic Composites network. “High-performance OCMC use almost exclusively Nextel 610 and 720 fibers from 3M [Minneapolis, Minn., U.S.]. Other suppliers have left the market, and Nitivy [Tokyo, Japan] fibers with 85%+ oxide content are still in development.”
But now, oxide fiber production is preparing to double, says Schüppel. Founded in 2008, and now part of the global association Composites United e.V. (CU, Berlin, Germany), Ceramic Composites has helped its members bring new CMC fibers to serial production. These include oxide fibers from RATH Group (Mönchengladbach, Germany) working with Fraunhofer ISC-HTL (Bayreuth, Germany) and also Saint-Gobain (Courbevoie, France) in collaboration with DITF (Denkendorf, Germany). Both groups are aiming to start continuous fiber production by 2024-25. BJS Ceramics (Gersthofen, Germany) started producing continuous SiC fibers in a pilot plant in February 2021, and has received investment from aircraft engine and components manufacturer ITP Aero (Bibao, Spain).
Another challenge is lengthy production times because CMC fibers and parts typically require multiple, high-temperature thermal cycles and process steps. However, global R&D is advancing new manufacturing technologies and also targeting sustainability. This article will explore some of these developments as well as CMC’s evolving applications and supply chain.
Though Germany has had significant CMC research for decades, the Ceramic Composites network aims to accelerate widespread implementation of new CMC technologies. It works to disseminate information, connect industry players for technology transfer and assist companies with government support and funding. “CU and Ceramic Composites have worked with our members to receive increased EU and German funding for a wide range of projects,” says Schüppel. The network published a CMC global market report in March 2023. This valuable resource — an abridged version is free — details commercially available fibers and producers, types of CMC, their processes and applications, as well as new developments from Ceramic Composites members. Parts of the infographic below were developed from this report, along with a position paper published in 2021.
Figure 1. CMC parts boost efficiency, enable energy transition. Burner lances made from Keramikblech oxide fiber/oxide matrix (OCMC) offer 10 times longer life versus metal alloy burner lances used in steam crackers (top). A hybrid OCMC furnace tube (alumina liner, metal insert, outer OCMC sheath) has successfully demonstrated electrical-powered steam cracking (bottom left). BASF Ludwigshafen has installed the world’s first large-scale electrically heated steam cracker plant, with a potential 90% reduction in CO2 emissions (bottom right). Photo Credit: Walter E. C. Pritzkow Spezialkeramik
Member Walter E.C. Pritzkow Spezialkeramik is a five-employee company near Stuttgart founded in 1994 by Walter Pritzkow after working with CMC at the German Aerospace Center (DLR). The company’s award-winning Keramikblech OCMC uses fabrics of Nextel 610 and 720 fibers and a hand layup process to create a wide array of parts. “We work mainly with industrial companies,” says Pritzkow, “such as Linde and BASF, but also with companies doing metal casting and heat treatment. We do small series of 10-100 parts but also larger production of parts like burner systems where we deliver 2,000 to 5,000 parts per year.”
Keramikblech currently uses mostly woven fabrics but also braided sleeves. The latter can be supplied affordably by a specialty braider in Germany in lengths up to 200 meters. Pritzkow explains the CMC production process: “We infiltrate the fabric with a water-based ceramic slurry using a doctor blade [squeegee] and then apply the wet fabric onto a special plastic mold. This is dried in a normal drying furnace at 60-90°C (for 4-5 hours up to 20 hours, depending on part thickness, size and complexity). We then remove the green body from the plastic mold and sinter it (1,100-1,200°C), typically overnight, in a standard sintering furnace. We machine the parts with diamond tools, and hole patterns in plates are laser cut.” Parts can be made and shipped in as little as 3 days.
But what about quality, and why not use SiC? “Because SiC/SiC and C/SiC are much more complex materials and require longer processing. My parts are easy to fabricate. Even though we don’t use an autoclave, like CHI [Composites Horizons Inc.] and COIC [COI Ceramics], I’ve compared our bending strength to theirs and found no difference. We use a matrix with alumina and zirconia (ZrO2) as a binder, which produces good preforms and CMC with good high-temperature properties. Our parts are typically used at 1,150-1,200°C; the maximum would be 1,280°C.”
The company also works with R&D centers and completes development projects for customers. “We are located near DITF and tested their oxide fibers in unidirectional and woven fabrics in a project with BASF,” explains Pritzkow. “The fibers they have developed are at the same level as Nextel fibers.” He is optimistic about Saint-Gobain’s industrial production of the fibers. “Nextel fabric is the most expensive part of our products,” he notes, “and delivery is a problem, often with delays of 3-6 months.”
Pritzkow sees a large potential for OCMC parts in the future. “More and more applications are being developed,” he says. “In the beginning, we worked with specialty companies and niche applications, but now large companies want to run thermal processes at higher temperatures. We have also worked with engine manufacturers and now have a project with Atmos Space Cargo [Rheinmünster, Germany] to develop a high-temperature fan using OCMC.”
Still, industrial applications will move much more quickly, says Pritzkow, “especially if oxide fiber costs come down. We’ll get more opportunities to replace metal parts which are destroyed in weeks and months due to coking and corrosion. OCMC parts provide a much higher lifetime — for example, our flame tubes last up to 4 years. Some say that our parts are too expensive, but companies like BASF have found the reduction in maintenance, shutdown and part replacement costs they provide are significant. Time without production is even more expensive. And now these companies can run for longer and at higher temperatures, so their overall efficiency is really benefiting.”
CMC was originally developed for rocket nozzles used in missiles and space launch vehicles in the 1970s. It expanded into thermal protection systems (TPS) for reentry vehicles and discs/rotors for aircraft brakes by the 1980s. Safran (Paris, France) claims it became the world leader in that technology and the first, in 1996, to qualify a CMC part for aeroengines. Its C/SiC outer flaps for the French Rafale fighter jet’s M88-2 engine were baselined for serial production, and more than 15,000 have been produced and used successfully. Safran continued developing CMC components (see “Ceramic matrix composite behavior enhancement for gas turbines hot section”) including its reported first test of a CMC turbine rotor in 2010.
GE is Safran’s partner in CFM International (Cincinnati, Ohio, U.S.) which produces the LEAP engine. GE began developing SiC/SiC engine parts in the 1980s. In 2014, it opened its CMC parts factory in Asheville, N.C., U.S., followed by its continuous fiber and prepreg plants in Huntsville, Ala., U.S. in 2018 (see “Vertically integrated CMC supply chain” and “Plant tour: GE Aviation, Asheville, NC, US”). GE’s fiber is based on the industry standard Hi-Nicalon-S SiC fiber produced since 1980 by Nippon Carbon (Tokyo, Japan). The technology was transferred via the joint venture NGS Advanced Fibers (Tokyo, Japan), formed in 2012 between Nippon Carbon (50%), GE Aerospace (25%) and Safran (25%). In 2021, GE Aerospace reported annual production of up to 10,000 and 20,000 kilograms of SiC fiber and prepreg, respectively, and had built more than 100,000 SiC/SiC high-pressure turbine stage 1 (HPT1) shrouds — 18 for each CFM LEAP engine — which had amassed more than 10 million hours in service. For the GE9X, it produces HPT1 shrouds and nozzles, HPT2 nozzles and the combustor inner liner and outer liner.
Also in 2021, GE Aerospace and Safran launched the Revolutionary Innovation for Sustainable Engines (RISE) program, which seeks a further 20% reduction in fuel consumption and emissions, centered on the team’s open fan design named for its absence of a case around the GE9X-sized turbo fan. Behind the fan is the core which holds the compressor, combustor and turbine, but as developed in RISE, it will be ultracompact — smaller than on a business jet, according to Mohamed Ali, VP of engineering for GE Aviation. In a June 2023 flightglobal.com article, Ali noted that HPT airfoils for the engine have benefited from CFM’s capabilities in CMC and were already manufactured and in testing using a modified military engine. RISE is on track for ground and flight tests by 2025 and flight tests using a hydrogen engine before 2030.
According to a GE blog in June 2023, some work for the ultracompact core has been completed through NASA’s Hybrid Thermally Efficient Core (HyTEC) project, which also began in 2021. Specific goals of that program include development of CMC liners for combustors, turbine blades and vanes as well as environmental barrier coatings (EBC). Meanwhile, GE’s XA100 Adaptive Cycle Engine developed for the F-35 fighter jet uses CMC more extensively than any commercial or military aeroengine to date, according to David Tweedie, general manager for advanced product at GE Edison Works. The engine reportedly delivers 25% better fuel efficiency, 10% better thrust and significantly more thermal capability compared to the current F135 turbofan from Pratt & Whitney (East Hartford, Conn., U.S.). GE began this development in 2007 with the Adaptive Versatile Engine Technology (ADVENT) program, where its prototype set a world record for the highest combined compressor and turbine temperatures, thanks in part to CMC. ADVENT also successfully tested SiC/SiC low-pressure turbine blades in an F414 turbofan demonstrator engine in 2015.
Development of CMC and UHTCMC has expanded significantly as the U.S. Department of Defense (DOD) seeks to counter threats from hypersonic weapons. Hypersonic speeds are not only reached by current long-range ballistic missiles, but also by reentry and space launch vehicles, like the SpaceX Falcon. The rapidly expanding New Space market is thus also driving new hypersonic technology.
Straddling both markets is Hypersonix Launch Systems (Brisbane, Australia) and its three hypersonic vehicles based on a patented hydrogen-fueled scramjet engine called Spartan that produces no CO2 emissions. Hypersonix states its mission is to provide reusable space launch technology that is sustainable. The company was founded in 2019 to commercialize 30 years of development by cofounder Michael Smart, whose research spans NASA Langley and the renowned Hypersonics Research Centre at the University of Queensland (Brisbane, Australia). In March 2023, Hypersonix was selected from 63 respondents to provide hypersonic vehicles for the DOD’s Hypersonic and High-Cadence Airborne Testing Capabilities (HyCAT) program, overseen by the DoD’s Silicon Valley-based Defense Innovation Unit (DIU).
The vehicle chosen by HyCAT is Dart. “It is designed to be an affordable, single-use test platform,” says Andy Mulholland, head of product for Hypersonix. “Its small size doesn’t allow for the equipment needed for recovery and reuse. But the next two vehicles are reusable: VISR, which stands for Velos intelligence surveillance reconnaissance, and the Delta-Velos Orbiter. Both will return to land on a runway. VISR is a hypersonic UAV [unmanned aerial vehicle] and Delta-Velos is our highly reusable and high-cadence solution for delivering small satellites to orbit.”
While Dart is fully 3D printed from Inconel metal alloy, VISR and Delta-Velos will need CMC to address the very high temperatures they will encounter at the faster speeds needed to access space and reenter Earth’s atmosphere, explains Sam Grieve, manufacturing lead at Hypersonix. “If we didn’t use CMC, we’d need an ablative TPS like other spacecraft.” Vaporized during reentry, ablative TPS must be re-applied, which adds complexity, weight and cost. High temperature CMC (HTCMC) is a better solution, he says, “to handle temperatures that are generated from friction with the air, but also from combustion inside the engine.” To that end, Hypersonix recently received a C/SiC demonstrator part that was designed in-house but produced, notes Smart, “using a very complex, never-done-before process working very closely over the last 2 years with a team of experts in Germany.”
Figure 2. Hypersonic HTCMC demonstrator. The Spartan scramjet engine (left) is integrated into the airframes of Hypersonix’ vehicles (above). The C/SiC combustion chamber below is not for flight, but to demonstrate the high-temperature ceramic matrix composite (HTCMC) manufacturing needed for future reusability and flight speeds up to Mach 12. Photo Credit: Hypersonix
The C/SiC part is a Spartan scramjet combustion chamber (Fig. 2) that is expected to handle temperatures up to 1,400°C. “It’s a manufacturing demonstrator that teaches us about the material and the technology,” says Grieve. “We’re hoping to eventually have these parts produced here by a local supply chain, but we may also produce some parts ourselves.” Mulholland notes it’s not a flight piece, “but is just to demonstrate that we could make the variable and intricate geometries needed. C/SiC was chosen as a more basic and well-understood HTCMC to start with, but eventually we’ll need much higher temperature materials.”
Where will these materials be used? “First for parts where combustion is occurring,” says Grieve, “and then other parts of the engine, followed by airframe leading edges that are directly exposed to hypersonic air flow.” Will Dart use a CMC combustion chamber in its Spartan engine? “Not in the engine,” says Grieve, “but we are planning for parts like leading edges. CMC technology is important for enabling reusability, but that’s not required for our first Dart vehicles. Our HTCMC demonstrator and development now is to prove out technology we’ll need as we move forward.”
The Dart’s first flight via the HyCAT program is planned for 2024 using Rocket Lab’s (Long Beach, Calif., U.S.) Hypersonic Accelerator Suborbital Test Electron (HASTE) rocket as a booster. Grieve explains why. “Spartan produces thrust as air enters through an inlet, gets compressed, then injected with fuel and combusted, which adds energy to the air flow, and then expands as it exits the nozzle. But unlike a turbojet, we have no moving parts — our compression is done by shockwaves which come off the front of the vehicle. The booster gets Spartan to the speeds above Mach 5 that it needs to operate.”
In August, Hypersonix announced an exclusive teaming agreement with Kratos Defense & Security Solutions (San Diego, Calif., U.S.). “This is complementary to our contract with DIU,” says Mulholland, “and gives us another channel for commercialization. Kratos also has a lot of experience in the space market and their Zeus rockets can also be used as a booster for Spartan.”
These first contracts are a key step in the company’s vehicle development. “As we continue to develop VISR and Delta-Velos, we’ll be running up against the limits of materials technology pretty quickly,” says Grieve. “There’s a nonlinear increase in temperature with Mach number, and our scramjet engine is designed to operate up to Mach 10, and we think even Mach 12. So, we’re going to reach pretty crazy temperatures sooner than later, and we’ll need to find and prove out new HTCMC and UHTCMC materials.”
A possible pathway for producing UHTCMC materials is spark plasma sintering (SPS), a process used to synthesize metals and ceramics by applying high amperage, pulsed direct current (DC) to powder in a sintering die (Fig. 3). Developed in the 1960s by Sumitomo Coal Mining Co., SPS was reported to achieve uniform, high densification in one-tenth to one-twentieth the time compared to conventional methods and use 60-80% less electricity. Modified to use a pulsed DC or AC power source, the process became known as field-assisted sintering technology (FAST) in the 1990s, according to FCT Systeme (Frankenblick, Germany), which claims it developed a novel process and the first FAST furnace in Europe in 2002. FAST reportedly enables the furnace to be “tuned” to the specific material being processed, which makes heating even more efficient than SPS.
Development has continued, with hundreds of SPS/FAST systems in use for R&D and commercial production. The Penn State Applied Research Laboratory claims FAST can be used to sinter CMC and metal matrix composites, achieving 100% density of near-net shapes at rates 70-80 times faster than conventional methods. In general, higher density enables higher mechanical properties.
Meanwhile, Safran announced in 2022 its investment in Sintermat (Venarey Les Laumes, France), citing its SPS technology that works with composites and biomaterials to produce complex shapes with a homogeneous microstructure. “This investment and our technology partnership will support Safran’s research and technology efforts,” said Eric Dalbiès, Safran senior executive VP of R&T and innovation, “especially concerning the materials and production processes for the components on tomorrow’s aircraft engines, reflecting the disruptive technologies needed to support the development of a more sustainable aviation industry.”
After years of CMC and UHTCMC materials and process R&D, MATECH (Westlake Village, Calif., U.S.), founded in 1989 by Ed Pope, began looking into FAST. Pope knew it worked great with powdered metals, ceramics and ceramic metal composites (cermets) but what about with continuous fiber CMC? “There was interest from the Office of Naval Research,” he says, “which is still pushing today for 2,700°F CMC for higher efficiency turbine engines. So, I talked to industry colleagues to see what had been tried. Their results were disappointing. The main approach was to begin with a 40-50% densified CMC and then use FAST, but what they ended up with was nowhere near 100% dense, and the properties were terrible because the fiber had been destroyed. I realized this approach was trying to get too much done in one step. The preforms had to be more dense to start with, down to 7-10% porosity. But that could be done with typical polymer impregnation pyrolysis [PIP], chemical vapor infiltration [CVI], melt infiltration [MI] or other processes. You then apply FAST. We demonstrated that this works, and we can get up to 99.9% dense SiC/SiC in less than 10 minutes with the strength and toughness we expect from a CMC.”
Fig. 3. FAST SiC/SiC parts. MATECH’s patented process (top) uses standard field-assisted sintering technology (FAST) equipment (bottom) which applies pulsed electrical current and a pressing force through a die into a CMC part, so that material reactivity and temperature increases through Joule heating. Photo Credit: MATECH, Fig. 2 from FCT Systeme Booklet FAST/SPS First Edition 2022
To prove the process works and measure critical properties, MATECH started with simple geometries including round discs and rectangular plates. More complex geometries, such as the aeroengine doublet vane shown in Fig. 3, are possible using graphite tooling within the FAST die. This was already designed for use with PIP but not yet used to create a FAST-densified part. The FAST process time, pressure and temperature for a shaped part is the same as a flat plate geometry.
Pope obtained two patents for this development: a process patent in 2019 and a composition of matter patent in 2020. “We were able to show we can reach near theoretical density for C/SiC and SiC/SiC composites,” he explains. “We have done limited testing with both types of CMC parts in rotational detonation engines at Wright Patterson [Air Force Research Lab, AFRL] that performed really well, but we haven’t made hot section blades or vanes to put in a test rig yet.” MATECH’s work so far has focused on precursor CMC made using PIP, which is highly amenable to complex shapes and fiber architectures. “We’ve found that 1,800°C was the sweet spot for this type of FAST processing of SiC/SiC CMC,” says Pope, “to achieve the density and fracture behavior we want.”
MATECH’s densification of SiC/SiC and C/SiC CMC has used between 30 and 100 megapascals of pressure. “This is a typical range used in FAST processing,” says Pope. “The electrical current used ranges between 2,500 and 10,000 amps, depending on sample size. But that is concentrated in a relatively short burst, which makes it much more efficient than hot-pressing techniques. Also, the heat is generated within the material itself — you’re not heating from the outside. By using electrical current and the pressure of the die, you’re efficiently adding thermal energy, but also vibrational energy which makes the material more reactive.” He adds that FAST opens the door to performance gains for hypersonic leading edge, nose tip and propulsion applications that were previously unattainable.
Founded in 2006, Free Form Fibers (FFF, Saratoga Springs, N.Y., U.S.) spent years working on continuous SiC fiber before pivoting its focus to production of fibers no longer than 1.5 inches. The move to discontinuous fiber was based on the science of CMC fracture toughening behavior, says CEO Shay Harrison. “Once you get past a fiber length-to-diameter ratio of 1,000:1, you don’t get additional fracture toughening. If we apply that ratio to our 30-micron-diameter fiber, we get a length of 1.25 inches.”
So, how does the FFF fiber compare to other SiC fibers on the market? FFF fibers are roughly 2X the diameter of the 10- to 18-micron diameters typical for spun polymer precursor fibers such as Hi-Nicalon-S and its competitors, says Harrison. “But our fibers have excellent handleability characteristics, such as being able to wrap them down to a radius of about 1/16 inch.” Fibers are also produced by using chemical vapor deposition (CVD) to deposit SiC onto a carbon or tungsten core, such as those made by Specialty Materials Inc. (SMI, Lowell, Mass., U.S.). “FFF fibers have no core, and thus, no dissimilar [non-SiC] material in the fiber structure,” says Harrison. “The SMI fibers also have a much larger diameter, on the order of 75-140 microns, which leads to processability issues when using them as reinforcements in CMC because of the high fiber stiffness.”
Figure 4. Laser CVD, scaling short SiC fiber production. Free Form Fibers’ patented single production tool (top) comprises 9 individual reactors, each using a multiplex laser to grow arrays of 50-100 SiC fibers up to 1.5 inches long (center), which can be used as nonwoven mats (bottom). Current gen2 production tools have 24 heads and gen3 production tools are being developed. Photo Credit: Free Form Fibers
How is the FFF process different from more conventional CVD? “Instead of heating up the entire volume inside a reactor and then introducing gases that deposit solid material onto a filament,” says Harrison, “we’re delivering the energy to decompose the gases in a focused laser beam onto a substrate, which initiates the breakdown of the gases to form solid SiC (Fig. 4). We’re basically growing the fiber by moving the substrate and the laser, to continuously deposit solid material.” Another difference is the reactor size. “It’s a little bit bigger than my fist,” says Harrison. “We have a very low CAPEX, but we use high-purity inputs and have developed our process to produce very high-quality fibers.”
CVD is indeed renowned for producing very pure SiC materials, but also for being glacially slow. “For typical hot wall CVD, the coating thickness onto the filament core drives the pull through rate of the fiber,” says Harrison. One industry source quotes production rates of 0.1 to 10 inches per minute for this process. “Yes, but that is for production of a single fiber,” notes Harrison. “We multiplex the laser so that we grow arrays of 50-100 fibers simultaneously. Even at the bottom end of that scale, we are producing 50 fibers at 1.25 inches long in approximately 10 minutes — which is effectively a production rate of more than 6 inches of fiber per minute. And we have scaled that to make kilograms of fiber per month. We then further scale by adding reactors, which are not expensive. So, our approach does have an economic basis to be not just competitive, but actually get to lower price points once we are at high-volume.”
FFF is maturing the technology now from lab to production scale. “We have built our first production tools, which are running 24/7 and we’re making material that we’re selling to customers. We’re on track to double our production capacity by the end of this year,” says Harrison. What are the targeted applications? “We’ve seen a lot of movement over the last year in ceramic-based additive manufacturing (AM), and that’s where having the discontinuous fiber has become an advantage. But we’re also looking at other material formats. We’re working with a partner to convert our fiber into a nonwoven mat, a format that is easily infiltrated with matrix, which reduces porosity, and also provides fibers in the Z-direction, which helps prevent delamination. We’re also developing relationships to look at turning our short fibers into a yarn that simulates continuous tow, in response to customers who still want that type of product.”
In addition to SiC, FFF is also looking into UHTCMC aimed at hypersonics applications. “One of these is silicon nitride (Si3N4) fiber for electromagnetic applications, which is desired but not yet available,” says Harrison. “We’re working through a DOD program to get that to a point of commercialization.” He notes that CMC is becoming a larger part of material solutions for an array of advanced applications, including nuclear energy, where the company is also active. “We want to be a manufacturer that supplies customers with real quantities of material for the higher end of temperatures in these applications,” he continues. “We think we have a good product that the market needs. We need to prove that and scale up, but we have good technology that has benefits and we’re going to be able to deliver that at a volume and price that matters to the CMC market.”
The National Composites Centre (NCC, Bristol, U.K.) has also seen increased demand for CMC. In August 2023, NCC announced its partnership with Lucideon (Stoke-on-Trent) to develop advanced CMC solutions. NCC will lead system and product design, plus industrial scale-up. Lucideon will provide analysis, evaluation and access to the new AMRICC Centre (Staffordshire), which it hosts and has developed with Stoke-on-Trent as a key part of the area’s “Ceramic Valley.” AMRICC includes the National Advanced Sintering Centre which focuses on flash, cold, microwave and SPS technology and includes a project to use novel sintering and AM to innovate CMC for aerospace engines.
U.K. aeroengine manufacturers do indeed support CMC development at NCC, including the AFP-CMC Core project with partners Rolls-Royce (London), Reaction Engines (Abingdon) and missile manufacturer MBDA (Stevenage). In 2022, NCC successfully demonstrated manufacturing of an oxide CMC part using automated fiber placement (AFP) of 3M Nextel prepreg (see “Leveraging towpreg to reduce the cost of CMC”). Follow-on goals included to optimize AFP parameters for more complex geometries and to investigate, with 3M’s help, wider material formats to reduce the number of joints between tape courses for higher performance.
NCC has also worked with universities to develop UHTCMC that can withstand temperatures up to 2,000-3,000°C and is working to mature extrusion-based 3D printing and other AM. Another key program is HASTE-F, where NCC and the UK Atomic Energy Authority (UKAEA) report they’ve developed a step change in SiC/SiC manufacturing that reduces cost to one-fifth that of current CVI methods while achieving 95% density (versus 75% for CVI) and shorter cycle times plus more complex geometries and thicker sections. NCC claims such SiC/SiC components have the potential to double the electricity generated from every gigawatt of heat produced compared to fusion reactors using advanced steel designs. This is possible because a SiC/SiC breeder blanket, and the coolant within it used to extract energy from the reactor, can withstand higher temperatures up to 900-1,000°C — at the upper end of current R&D metal breeder blankets. This allows more efficient generator approaches to be used. NCC adds that it is pioneering industrialization of U.K. SiC/SiC design, modeling, manufacturing and technology capabilities to support the STEP (Spherical Tokamak for Energy Production) program aiming to construct the first grid-connected fusion reactor by 2040.
The CEM-WAVE project (2020-2024) aims to produce novel CMC parts using a microwave-assisted chemical vapor infiltration (MW-CVI) process to reduce production time and cost. For example, the University of Birmingham in the U.K. reduced multiple cycles totaling 1,000 hours to a single 100-hour cycle for SiC/SiC parts. CEM-WAVE has produced tubular preforms using filament winding of Nextel 610 fiber with an aluminum phospate (AlPO4) coating and a zirconium matrix which will be sintered in equipment modified by Archer Technicoat Ltd. (ATL, High Wycombe, U.K.). Coatings have been developed by Fraunhofer ISC (Bayreuth, Germany) to help the largely microwave-transparent alumina fibers and matrix to absorb microwaves.
Fraunhofer IKTS (Dresden, Germany) has developed a fused filament fabrication process for ceramics (CerAM FFF) which uses homogeneous, highly filled thermoplastic filaments with a diameter of 1.75 millimeters. They are melted and deposited by the printhead and and then densified using PIP to form the CMC. This process offers the possibility to integrate ceramic fibers into the filaments, enabling AM of short to long fiber-reinforced CMC. A variety of ceramic matrices have been demonstrated and SiC/SiC components are in development.
Photo Credit: DITF (German Institute for Textile + Fiber Research)
Having developed oxide fibers since 1990, DITF is now in partnership with Saint-Gobain for the industrial production of alumina (OxCeFi A99) and mullite (OxCeFi M75) fibers, scheduled to start in 2025. DITF fiber R&D continues, aiming at even better properties using multi-phase systems and elements such as Zirconium (Zr) and Yttrium (Y), with pilot production of Zr-toughened alumina (OxCeFi ZTA) and Zr-toughened mullite (OxCeFi ZTM) fibers already well advanced.
The C3HARME project (2016-2020) aimed to develop novel UHTCMC materials for hypersonic and space applications. Coordinated by CNR-ISTEC (Faenza, Italy), the project included partners such as Airbus, Ariane Group, Avio, DLR and others. Subscale rocket nozzles using short and long carbon fiber were fabricated and densified using SPS for densification and tested to technology readiness level (TRL) 6. TPS plates were also made using 2.5D carbon fiber preforms impregnated with zirconium diboride-alumina (ZrB2) slurry and converted using radio frequency-assisted CVI. Such TPS was tested to TRL 5. The C/ZrB2 UHTCMC materials developed in C3HARME were patented by CNR-ISTEC and have been commercialized by spinoff company K3RX.
UHTCMC nozzle and plate. Photo Credit: K3RX
Fraunhofer IKTS produced SiC/SiC parts using filament winding with an integrated laser system for sintering. This eliminates the need for post-winding sintering in a furnace. “We showed the feasibility of the laser process and built a simple CMC tube as a demonstrator. However, the part strength was not sufficient for industry applications,” says Clemens Steinborn, testing laboratory manager and researcher at Fraunhofer IKTS. “We are pursuing funding for a follow-up project to reach a higher TRL.”
Photo Credit: University of Bayreuth
The University of Bayreuth is researching the production of oxide CMC using short fibers (14-60 millimeters long) in the FlexFiber project (2023-2026), funded by the German Research Foundation (DFG). The goal is a robot system that can be operated flexibly and intuitively by technicians.
Another key CMC technology being developed in the U.K. is continuous coating of SiC fiber. “There are three main parts to a CMC — the fiber, the interface coating and the matrix,” explains John Yeatman, managing director of Archer Technicoat Ltd. (ATL, High Wycombe, U.K.). “For nearly all CMC, at the moment, the interface coating on the fibers is produced using CVD. Typical coatings include boron nitride, silicon nitride or a plain carbon interface.” ATL has provided advanced coating solutions for 40 years and interface coating for CMC for 20 years. “We perform R&D, design systems, build and sell coating equipment and also provide coating services,” says Yeatman. “Most of the latter has been using batch processes, where you take your woven fiber, preform or layup and put it in a mold, and then you’ll infiltrate it using CVI and put the coating on that way. We say CVI instead of CVD if we’re coating all of the surfaces in a porous preform.”
“But there are also continuous coating methods,” Yeatman continues, “where you coat the fibers before layup. As long as you can make the coating both stable and flexible — so that you can handle the coated fiber and lay it up — then it has obvious advantages in efficiency. Pioneered mostly by GE, this is used in its industrial-scale CMC production. But that technology is restricted, so nobody else in the world has access to it.” Yeatman says ATL’s goal is to develop continuous coating capability for CMC fibers that can be used by pretty much anyone, especially companies outside the U.S.
This was the goal for the Continuous Interface Coating for Silicon Carbide composites (CICSiC) project, led by ATL. “The CICSiC project allowed us to design and build a prototype piece of coating equipment, operate it and test it. And it’s been very successful,” says Yeatman. The U.K. project partners included the University of Sheffield Advanced Manufacturing Research Centre (AMRC, Rotherham), Cygnet Texkimp (Northwich) and TISICS (Farnborough). “Cygnet provided the fiber handling expertise that could help us run the fiber back and forth through the furnace,” says Yeatman. “You have to control the tension and speed, which is quite tricky, because the fibers are very fine, easily abraded and broken.” TISICS also provided fiber handling expertise and helped design a specific aspect of the coating equipment, while AMRC tested the flexibility of the coated fiber.
With CICSiC completed, ATL is seeking a follow-up project to accelerate the final development needed. “Although the machine works well, and we’re using it, you can only put a single coating on when you pass the fiber through the furnace,” explains Yeatman. Multiple coatings are typical, he adds, and used by GE, for example. “Each layer has a separate function, be it as a diffusion barrier, debonding [good not great interface] or oxidation resistance. For now, if you want a multi-layered coating, you have to wind it through one way and then wind it back through the other way. So, we need to improve our fiber handling for this and also to enable longer runs. We want to get to a point where we can build a piece of equipment with multiple coating stages that we can sell, so our customers will be able to produce their own coated fibers.”
Customers interested in this technology include the major aerospace companies outside of the U.S. and a growing supply chain to produce SiC fibers in Europe. “But there is now an operation in Germany and one of the ideas is to basically add coating as a part of the fiber production process,” says Yeatman. “Just put a CVD system on the end, so that as the fiber comes off the production line, it gets coated, continuously straight away. And to me, the potential that could have has already been proven.”
When asked what is needed to increase the use of CMC, Ceramic Composites network’s Schüppel says it varies by each of the four major types. “Industry is looking for reactor tubes that also have good thermal shock resistance. For Ox/Ox, the challenge is to get CMC parts dense enough to hold gases.” For SiC/SiC, he notes GE has fiber, coating and parts production all in-house. “The challenge is that European SiC fiber producers don’t necessarily have that know-how in coatings and their interaction with the SiC matrix nor in the intricacies of CMC parts production.” To expand C/SiC applications, says Schüppel, “simulation is needed to create a virtual twin along the entire process chain. This will help reduce trial and error, and thus cost. For C/C, aircraft brakes are the largest market. Safran has said it is researching new CVI. The challenge is to get high-quality parts but also meet targets for less energy, cost and carbon footprint.”
Indeed, sustainability of CMC is a nascent, but growing topic. For a discussion of energy use, lifecycle analysis and bio-based materials, watch for our upcoming digital article: “Increasing the sustainability of CMC.”
Spirit AeroSystems was an established aerospace supplier when it earned that distinction, winning the contract for the Boeing 787’s Section 41. Now its sights are set on the next generation of aircraft.
Compared to legacy materials like steel, aluminum, iron and titanium, composites are still coming of age, and only just now are being better understood by design and manufacturing engineers. However, composites’ physical properties — combined with unbeatable light weight — make them undeniably attractive.
The matrix binds the fiber reinforcement, gives the composite component its shape and determines its surface quality. A composite matrix may be a polymer, ceramic, metal or carbon. Here’s a guide to selection.
Oven-cured, vacuum-bagged prepregs show promise in production primary structures.
New facility in Germany is part of regional supply chain and vision to lead green energy transition using CMC, supported by German government and Composites United’s Ceramic Composites network.
GE Aviation's new ceramic matrix composite supply chain includes fiber, prepreg and unprecedented parts production facilities, plus a strongly tied 4-bump development stream.
An avant-garde approach to unprecedented CMC parts production.
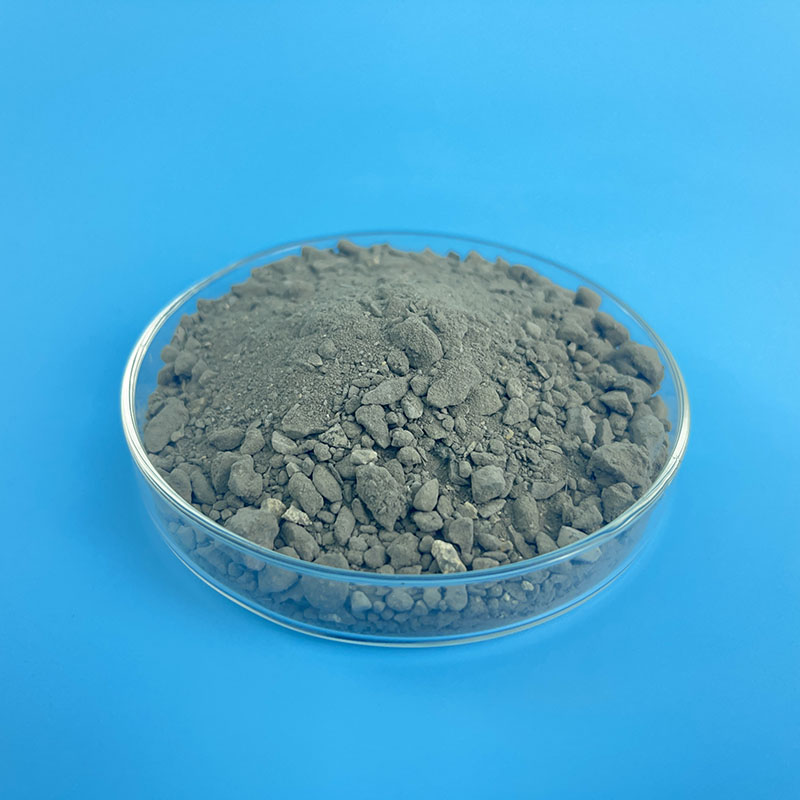
High Alumina Castable Refractory CompositesWorld is the source for reliable news and information on what’s happening in fiber-reinforced composites manufacturing. Learn More